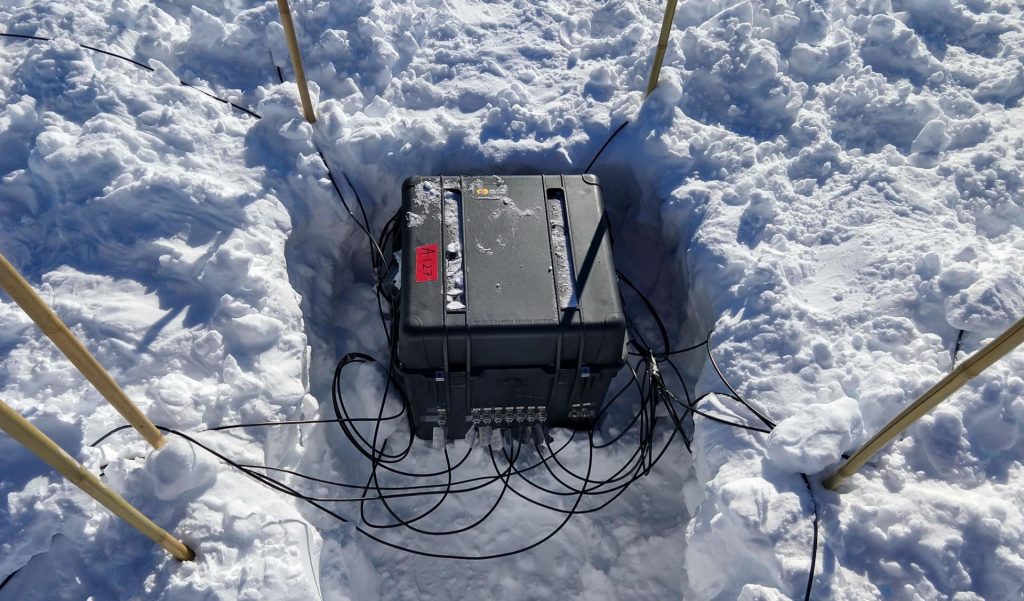
Overview
The neutrino is one of the most elusive particles in our Universe. It carries almost no mass, it carries no charge and it only rarely interacts with matter. We are all bombarded with neutrinos every day without noticing any effect.
To detect neutrinos, very large experiments are needed – preferably having several tons of detector target material. Examples of such targets include water-filled mines in mountains (such as in Japan) and vast volumes of ice (such as IceCube at the South Pole)
Neutrino detectors have been getting bigger and bigger over the years as scientists attempt to measure ever more rare neutrino signals. Neutrinos come in different energies and in general it holds that the higher the energy, the rarer the neutrino.
The larger a detector gets, the more likely it is to catch a very rare event. At some point, simply increasing the size of a detector becomes too costly and new technologies have to be investigated to gain in detector size while keeping the costs at bay. Scroll down below to learn more!
ARIANNA Stations
ARIANNA targets neutrinos of energies higher than ever detected before. The design utilizes the fact that a rare neutrino interaction in ice causes light emission in certain materials (the most common detection technique so far) and generates a radio pulse.
In clear ice, radio pulses are detectable over several kilometers, which allows the instrumentation of large quantities of ice with relatively few radio detectors.
How It Works
At Moore’s Bay in Antarctica where ARIANNA is located, an additional characteristic of the ice is present. The Ross Ice-Shelf is about 500 meters thick and has a very smooth bottom surface. The ice-water surface acts like a mirror for radio waves. Radio pulses in the ice are reflected up towards the surface. So the detector antennas do not have to be buried to see the signal but can be placed at the surface, which makes installation and deployment much easier and less costly.
Currently, eight ARIANNA stations are taking data on the Ross Ice-shelf, in a prototype experiment called the Hexagonal Radio Array (HRA). According to its initial design, the full ARIANNA telescope will contain about 1000 detector stations spread over an area of 36 km by 36 km.
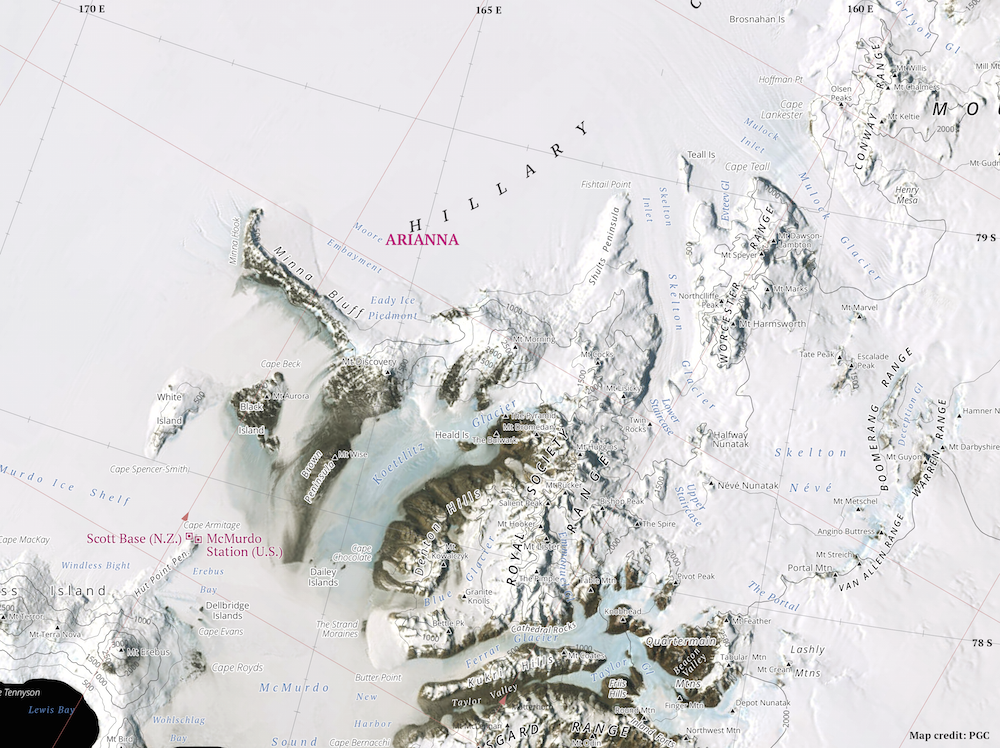
Our Why
There are several purposes for detecting neutrinos of so-called “cosmogenic energies”. It is known that cosmic rays (charged particles such as protons and atomic nuclei) bombard the Earth with energies higher than any man-made machine has been able to achieve so far.
As of 2016, it is still unclear what sorts astronomical objects can accelerate these particles to such energies. Candidates include super-massive black holes, Galaxy clusters, Gamma Ray Bursts, and other violent objects in the Universe. The sources have not been identified since it is difficult to trace cosmic rays back to their origin. Cosmic rays are charged particles and are therefore deflected in magnetic fields, which means that their arrival directions on Earth do not necessarily point back to the source.
Due to the interaction of cosmic rays with the cosmic microwave background, ultra-high energy neutrinos are expected to be produced alongside cosmic rays. These neutrinos are more difficult to detect than cosmic rays but do fly in straight lines. Thus, the detection of a cosmogenic neutrino would point right back to the neutrino’s source and help unravel the mystery of the origin of ultra-high energy cosmic rays. Such a multi-messenger approach will teach us more about the mechanisms in astronomical objects and is needed for a complete picture of the physics at work.

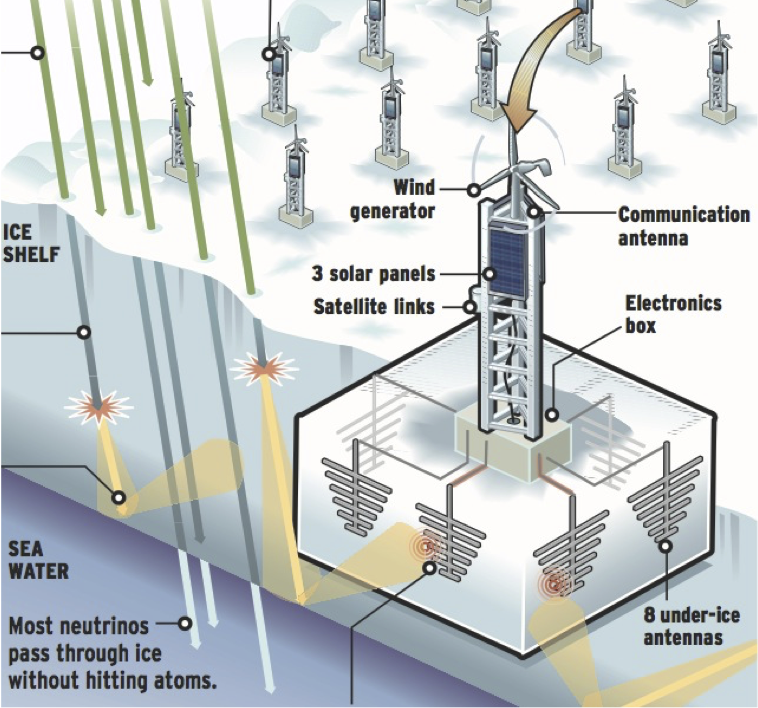
Goals and Impact
In addition, there might be objects in the universe that directly produce neutrinos of very high energies. While there is evidence that neutrinos of cosmic origin are out there, finding a neutrino source would be an exciting discovery and would open the door wide for neutrino astronomy. In addition, the elementary physics of neutrinos at such high energies is unknown. Detecting them in ARIANNA will allow for studies of fundamental parameters such as cross-sections, which cannot be measured at present with man-made accelerators.
The ARIANNA collaboration has shown, with its pilot array, that the radio technique has potential and that a larger array will be able to detect cosmogenic neutrinos if they are indeed out there. Since the HRA is currently a small array, its sensitivity to neutrinos is also still low and no detection has so far been made. In the figure below, this is indicated by a limit on the flux of neutrinos that could have been detected by the HRA. The shaded regions indicate where we would expect a signal from cosmogenic neutrinos (different shading indicates different theoretical models). The dashed lines indicate what future arrays might be able to detect, including the full ARINNA telescope of 36 by 36 kilometers.